Bridging the Gap Between Biotech Patents and Commercialization
- Guru Singh
- 7 days ago
- 23 min read
Updated: 2 days ago
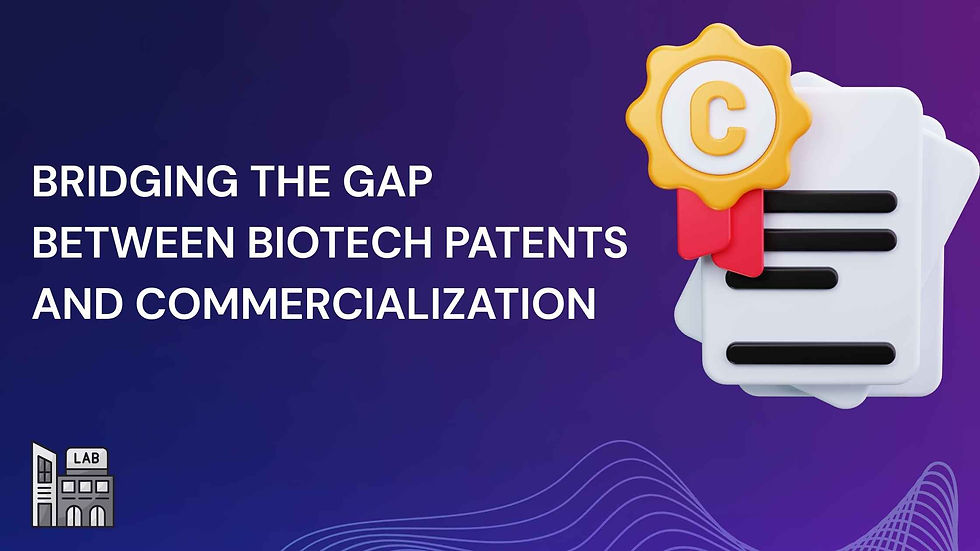
In the Lab, But Not Yet in Life: Biotech research is producing remarkable discoveries and patents, yet too few are becoming real-world products. This disconnect between invention and impact is a central theme discussed by Guru Singh (Founder & CEO of Scispot) and Kevin Chen (CEO of Hyasynth Bio) on the "talk is biotech! with Guru Singh" podcast. Scispot, known for offering an advanced AI-driven tech stack for life science labs, often works with innovators at the cutting edge of biotech. Singh and Chen observe that while laboratories churn out patents, commercialization lags behind, leaving potential value on the table. As they urge, government funding should "prioritize commercialized biotech solutions over mere patents," rather than measuring success by patent counts alone. Their vision is to transform groundbreaking science into tangible products that benefit industries and lives.
The Chasm Between Invention and Impact
Biotech has always walked a precarious path from the eureka of discovery to the reality of a marketable therapy or technology. It's common for promising research to languish in what industry insiders dub the "valley of death," the yawning gap between lab benchtop and marketplace. Decades ago, this gap was stark: by the late 1970s, the U.S. government had amassed an estimated 30,000 patents from federally funded research, yet only around 1,200 (4%) were ever licensed out, and even fewer made it to market. In other words, patenting an invention hardly guaranteed its use. A recent U.K. government analysis reinforces this point: "IP registration is a flawed indicator of innovation success... even if a patent is filed, that does not necessarily mean the invention will be used". In biotech especially, a patent is just the starting line. What lies beyond, product development, clinical trials, regulatory approval, manufacturing, distribution, is an arduous marathon requiring resources and know-how beyond the scope of academic research.
Singh and Chen highlight this disconnect from a Canadian perspective. Canada produces world-class biomedical research and plenty of patents, but often sees the economic rewards flow elsewhere. The discovery of insulin is a famous example: Canadian scientists discovered insulin, yet the rights were quickly licensed to Eli Lilly in the U.S. and Nordisk in Denmark, paving the way for what became Novo Nordisk, a pharma giant that today contributes billions in taxes to Denmark. "For decades, Canada has watched its life-saving discoveries drift across borders, generating billions for foreign enterprises, while Canadian coffers remain comparatively empty," notes one analysis. This story repeats in many countries when innovations aren't commercialized domestically. Even the U.K., home to Nobel-winning science, struggles in what McKinsey calls "Translation and Impact, converting research into intellectual property and commercial products." In short, there is often a mismatch between biotech innovation and commercialization capacity. Addressing this mismatch is critical not only for economic returns but for societal benefit, after all, a patent sitting on a shelf cures no disease and creates no jobs.
Structural and Financial Barriers to Commercialization
Why is it so challenging to turn biotech patents into products? The obstacles are structural, financial, and cultural:
Long, Costly Development Cycles: Bringing a biotech innovation (say a new drug or biotech tool) to market can take 10+ years and hundreds of millions to billions of dollars. This daunting timeline scares off many investors until a technology is well-validated. Early-stage startups face a funding "valley of death" where academic grants have run out but commercial investors aren't ready to step in. As Kevin Chen describes, an aspiring biotech founder doesn't necessarily need to "quit everything" immediately; they can "use what's out there, get to know the industry, and build it up till ready to dive in". However, not all scientists have the means or mentorship to do so, and many promising projects stall without bridge financing. Traditional venture capital often prefers software or tech sectors with quicker returns, leaving biotech innovations in a funding lurch unless special mechanisms exist (more on those in a moment).
Infrastructure and Translational Gaps: Academia and industry operate in different worlds. Academic labs excel at discovery and publishable findings, but turning those findings into a manufacturable, regulated product requires expertise in engineering, clinical trial design, regulatory affairs, and scale-up. These translational skills and facilities (e.g., GMP manufacturing labs, incubators, mentorship from industry veterans) may be lacking. Tech-transfer offices in universities try to bridge this gap, but their success varies. In the U.K., despite a strong science base, CEOs note an "uphill battle for talent" and a lack of the "close-knit [biotech] community" that helps U.S. startups grow. Structural support, such as incubators, accelerators, or partnerships with pharma, is needed to guide inventors through the complex pipeline.
Incentive Misalignment: Academic researchers are typically rewarded for publications and patents, not for nurturing a business. Government grants likewise often measure outputs like papers or filed patents. Guru Singh points out that "doing research has its role to play, but when it comes to industry, it would be nice if government funding really focused on impact", i.e., whether the research leads to a real product, rather than only the research itself. Currently, a professor might secure funding to discover a drug target and file a patent, but there is less support for taking it further. The result is that many patents sit unutilized, or get licensed cheaply, because neither the inventor nor the funding system is geared toward commercialization. In some cases, too, regulatory and policy hurdles can be structural barriers. For example, if drug price controls or healthcare reimbursement are very restrictive, companies may be hesitant to launch products in that market, reducing incentive to develop homegrown innovations.
Talent and Culture: Biotech commercialization requires interdisciplinary teams, not just brilliant scientists, but also business leaders, regulatory experts, and operations specialists. In emerging biotech ecosystems, there may be a shortage of experienced entrepreneurial talent. Biotech is often called a "team sport," where success depends on bringing together people with diverse skill sets to tackle science and business challenges. In regions without a strong history of biotech startups, academic founders might not know where to find co-founders or advisors who have taken a product to market. Culturally, there can be risk-aversion: top graduates may opt for stable jobs in academia or established companies rather than the uncertainty of a startup. This conservatism can limit the formation of new ventures needed to commercialize IP.
Financial Risk and The "First Customer" Problem: Even when seed funding is obtained, biotech startups face steep costs in later stages (e.g., conducting clinical trials or building manufacturing). Investors capable of funding these stages (larger VCs, corporate partners) often want to see proof that the innovation works and that there's a market. Without an existing commercial track record, it's a catch-22. Government or strategic customers can play a role as "first customers" or funders to validate technology (as, for instance, the U.S. Defense Department often does for biotech in defense/health emergencies). Lacking that, many startups from smaller markets feel compelled to relocate or partner abroad to access capital and markets, exacerbating the home country's loss of economic benefit. This is why observers emphasize that "innovation without commercialization is a path to lost opportunity": without a strategy to cross the finish line, the initial innovation can be effectively lost.
In sum, the journey from patent to product is fraught with what Singh calls "research and real-world impact" gaps. Overcoming them requires smart support systems, which some regions have developed more extensively than others. Let's examine how various biotech-heavy regions, Canada, the United States, the United Kingdom, Germany, and Singapore, are grappling with this challenge, and what lessons they offer.
Global Perspectives: How Leading Regions Bridge the Gap
No two innovation ecosystems are identical. Different countries have taken different approaches to bridging the lab-to-market gap in biotech. Below is a comparison of commercialization support structures across several biotech hubs, followed by specific examples and insights:
Commercialization Support Structures in Key Biotech Regions
United States:
University IP Policy: Bayh-Dole Act (1980) gives universities ownership of inventions from federal funding, spurring tech-transfer offices. Academic entrepreneurship became mainstream thereafter.
Early-Stage Funding Support: SBIR/STTR grants (> $3B/year) provide non-dilutive funding to small biotech firms. Agencies like NIH also run I-Corps bootcamps to train scientists in commercialization. A vibrant venture capital ecosystem invests ~$36B/year in biotech (world's largest).
Incentives & Tax Policies: R&D tax credits support research. No patent box federally, but strong IP protection environment. Orphan drug and other FDA incentives encourage niche biotech innovation. U.S. government procurement (e.g., BARDA contracts for vaccines) can de-risk commercialization by serving as first buyer.
Translational Infrastructure: Extensive incubators and accelerators (e.g., J&J JLABS, IndieBio) in biotech hubs. NIH translation centers and public-private partnerships (e.g., CARB-X for antibiotics) help advance lab discoveries. A culture of academia-industry collaboration (often scientists-turned-entrepreneurs) plus large biotech clusters (Boston, SF) create networks that ease the path to market.
Canada:
University IP Policy: Universities and research institutes generally own or share IP with inventors (Bayh-Dole-like policies in place). However, many patents end up unused or licensed abroad. Singh and Chen note Canada must "turn groundbreaking science into tangible solutions", keeping IP benefits onshore.
Early-Stage Funding Support: NRC-IRAP grants and provincial programs provide seed funding to startups. Strategic Innovation Fund invests in scaling life-science firms. Despite these, Canada's funding often skews toward early research; later-stage ("mezzanine") capital is scarce. This gap forces firms to seek U.S. investors or relocate. The government is ramping up support (e.g., a $1B Venture Capital Catalyst and Life Sciences fund) to fill this gap.
Incentives & Tax Policies: Generous R&D tax credits (SR&ED) rebate a portion of research expenditures, aiding biotech startups' cash flow. Canada is exploring a "patent box" tax incentive to reduce tax on income from intellectual property commercialized in-country. Quebec province already introduced an IP tax incentive (4% tax rate on patent-derived income) to retain pharma and biotech companies.
Translational Infrastructure: Tech incubators and translational centers are emerging (e.g., adMare BioInnovations in Montreal, which helps develop academic discoveries into companies). However, the ecosystem is still maturing. Clinical trial infrastructure is being bolstered by government strategy (recognizing that lack of domestic trials stalled many innovations). Canada's SynBio communities and initiatives like Stem Cell Network also try to bridge academia and industry.
United Kingdom:
University IP Policy: Historically, the British Technology Group had a monopoly on academic IP until reforms in 1985 liberated universities to manage their own patents. Now top universities (Oxford, Cambridge, etc.) actively file patents and launch spin-offs. The "Golden Triangle" universities have professional tech-transfer offices and often take equity in startups.
Early-Stage Funding Support: Innovate UK (government innovation agency) offers grants (e.g., Biomedical Catalyst) for proof-of-concept and commercialization of biotech research. The NHS (health service) supports innovation through programs that pilot new technologies. The U.K. also has growing venture capital investment, biotech startups raised $3.6B from 2018-2020, a 20% jump over prior period, though still far behind U.S. levels.
Incentives & Tax Policies: The UK Patent Box regime offers a 10% corporate tax rate on profits from patented inventions, to incentivize companies to commercialize IP in the U.K. (in place since 2013). R&D tax credits and the Enterprise Investment Scheme (angel investor tax relief) encourage biotech investment.
Translational Infrastructure: Catapult Centres, e.g., Cell and Gene Therapy Catapult, are government-funded translational institutes that provide expertise and facilities to help move innovations to market. Strong regional clusters (London-Oxford-Cambridge, Scotland, etc.) provide incubators, shared lab spaces, and mentorship networks. Despite this, a McKinsey report finds the U.K. still "struggles converting world-leading science into commercial products", indicating ongoing effort needed in translation and scale-up support.
Germany:
University IP Policy: Academic inventors historically had "Professor's privilege" (full control of their inventions) until a 2002 law aligned with Bayh-Dole principles (universities now typically own patents, with revenue-sharing to inventors). Public research organizations like Max Planck Society and Fraunhofer Society actively patent discoveries, Fraunhofer focuses on applied research and licensing to industry.
Early-Stage Funding Support: The federal government has long used targeted programs: BioRegio (1995) was a competition that fostered biotech clusters and tech-transfer offices. GO-Bio (since 2005) provides grants to life scientists to start companies from their research. The state-backed High-Tech Gründerfonds (HTGF) seed fund co-invests in biotech startups (often being first-money-in). Germany also benefits from strong industrial players and family offices investing in biotech (as seen in BioNTech's rise).
Incentives & Tax Policies: Generous public R&D funding (Germany invests ~3% of GDP in R&D, among the highest) and innovation incentives. While Germany doesn't have a patent box yet, it offers R&D tax subsidies and regional incentives. Notably, the government in the 1990s even absorbed 90% of losses for private VC investors in startups to encourage funding, a bold incentive that led to a startup boom. (The policy was later dialed back after the dot-com bubble.) Today, discussions continue on new incentives to keep scale-ups in-country.
Translational Infrastructure: Fraunhofer Institutes act as bridges between academia and industry, with 76 institutes working on applied R&D and often developing prototypes that companies can commercialize. The pharma industry and universities collaborate via cluster initiatives (e.g., Munich's biotech cluster). There are numerous science parks and incubators (like Martinsried in Munich or Berlin-Buch) where startups co-locate with research institutes. Germany's emphasis on engineering and manufacturing means strong support for process scale-up (biomanufacturing), which is crucial for commercializing biotech. Recent successes like BioNTech and CureVac (both spun out of academia) underscore improvements in translating science to products, though these successes were aided by sizable public and private investment.
Singapore:
University IP Policy: A highly state-driven model: Singapore's Agency for Science, Technology and Research (ASTAR) often holds patents from its research institutes and actively encourages researchers to commercialize. Unlike environments where academia is hands-off about business, ASTAR's policy has been pro-entrepreneurship: "Knowing that successful biotech companies generate both revenue and jobs, the government encourages researchers to apply for patents and, based on those patents, to launch start-ups, offering three-year leaves of absence and S$150k seed money as incentive." This proactive stance has led many foreign scientists and locals to spin out companies with government support.
Early-Stage Funding Support: The government commits substantial funding to biotech. Researchers in Singapore can tap grants specifically for translating research (e.g., Translational R&D grants). There are co-investment programs where government-linked entities (like EDBI, the VC arm of the Economic Development Board) will invest alongside private VCs in promising biotech startups. Singapore also partners with foreign venture firms, for instance, a recent A*STAR partnership with Flagship Pioneering (the U.S. VC behind Moderna) pools S$100M to launch biotech companies in Singapore. This effectively imports commercialization expertise.
Incentives & Tax Policies: Singapore offers tax incentives such as the Development and Expansion Incentive for companies investing in emerging industries, and has low corporate tax rates. While it doesn't have a patent box per se, it often gives tax holidays or grants to IP-rich companies setting up regional HQ or manufacturing in Singapore. The government's approach is hands-on: they will fund infrastructure and even construction of facilities for companies willing to localize operations.
Translational Infrastructure: A concentrated innovation hub, Biopolis, co-locates research institutes, hospitals, and biotech companies to stimulate collaboration. ASTAR's tech transfer arm (Accelerate) manages thousands of patents and has licensed hundreds to industry. Major pharma companies have been attracted to set up research centers there, providing local startups opportunities for partnerships or talent access. The ecosystem is smaller but tightly integrated, with the government often acting as matchmaker between research and industry. Singapore's explicit goal is to be a biotech commercialization hub in Asia, and it has seen successes (e.g., homegrown vaccine and medtech startups) though it continues to invest heavily to stay competitive.
As above suggests, each country has unique levers to pull. The United States has been a trailblazer with the Bayh-Dole Act, a framework that "catalyzed a surge of interest in commercializing academic research" by clarifying IP ownership. Crucially, the U.S. also created the SBIR program in the 1980s, which has since become "one of the most emulated government R&D programs in the world," adopted in spirit by countries from the U.K. to India. SBIR's success stems from aligning incentives: it gives researchers funding and a mandate to pursue commercialization, often yielding spin-off companies and even FDA-approved products from what began as academic ideas. The U.K. and others have launched similar small-business grant programs (e.g., the U.K.'s Small Business Research Initiative, modelled after SBIR).
Canada's approach, while rich in research support, is now recognizing the need to tilt the balance from "patent" to "product." Guru Singh and Kevin Chen's discussion comes as Canada has launched its Biomanufacturing and Life Sciences Strategy, investing billions to shore up everything from talent to manufacturing plants. Notably, Canada's industry association BIOTECanada has been advocating for a Patent Box regime to "ensure companies do not locate IP in other countries" and instead grow domestically. The awareness is there: without strong pull for commercialization at home, Canadian innovations will continue to enrich others. Singh and Chen specifically call on funding agencies to reward impact, e.g., if a biotech project can show a path to a new vaccine or a bioproduct for industry, that should perhaps weigh more than just publishing another paper.
Germany and Singapore illustrate two different but effective models. Germany, through sustained public-private efforts (fraunhofer institutes, public venture funds, etc.), built an industrial biotechnology base. It learned in the 1990s that throwing money at startups can yield many new companies (startup rate shot up fourfold), but sustaining them to global success required patience and global integration. Germany's recent biotech stars (like BioNTech) benefited from decades of research funding, tech-transfer support, plus entrepreneurial scientists willing to work with investors, and importantly, flexible funding in the right moments (the founders of BioNTech received significant state grants early on, and later private capital).
Singapore, on the other hand, used a highly directed strategy: invest in people and facilities, "get the best to come here", and support scientists to become entrepreneurs by giving them funding and time off from academia. This has rapidly built a nascent biotech industry in a country with no prior track record in pharma. The trade-off is heavy government spending and involvement, which Singapore deems necessary to bootstrap local capacity.
The common thread across these regions is that bridging the gap takes more than a laissez-faire approach. It requires deliberate frameworks and investments: whether through laws that encourage tech transfer, funding programs that de-risk early commercialization, tax policies that retain IP, or institutions that physically connect lab research with industry expertise. With those in place, inventors are more likely to take the plunge into entrepreneurship, and private investors are more likely to back them.
Government Funding: Moving from Patents to Impact
One of the strongest levers to close the lab-to-market gap is government funding strategy. Governments pour billions into basic research, which is fundamental, but how they allocate support down the line can make or break commercialization:
Reorienting Metrics and Incentives: If grant success is measured by papers and patents, that is what researchers will optimize. Shifting the focus to impact doesn't mean abandoning basic science; it means adding programs that specifically back the translation of promising research into applications. For instance, the U.S. National Institutes of Health created Centers for Accelerating Innovation and translational science awards to help researchers develop drug candidates further than a typical grant would. Guru Singh suggests governments should ask, "Does this funding help turn an idea into a product?" and allocate at least a portion of budgets to those projects that answer yes. This could involve milestone-driven grants where teams must reach prototyping, proof-of-concept in animals, etc., rather than stop at publication. The U.K.'s Industrial Strategy and Horizon Europe have started moving in this direction, tying funds to "impact plans" and requiring collaboration with industry partners for certain grants.
Balancing Patents with Open Innovation: Not every innovation needs a patent, and not every patented discovery will be commercialized. The U.K. IPO study we cited earlier explicitly warns against treating patent counts as proxies for success. Governments can encourage a healthy balance by supporting patenting when it's useful (e.g., providing IP management help to academic startups) but also encouraging other routes like open licensing for platform technologies that can spawn ecosystems (similar to how some NIH discoveries are licensed non-exclusively to many companies, accelerating their use). The key is to ensure IP is not a dead-end. If an academic patent is sitting unlicensed, could funding agencies intervene? Some ideas include "second look" programs where after a few years an unlicensed patent is reviewed for inclusion in a national commercialization program or put into patent pools that startups can tap at low cost. The overarching goal: avoid patent graveyards, maximize innovation diffusion.
Bridging Programs and Funds: As highlighted, programs like SBIR (Small Business Innovation Research) in the U.S. provide critical early funding with the explicit aim of commercialization. These effectively act as bridges over the valley of death by supplying ~$150k-$1M in Phase I/II grants to small companies to do feasibility and development. Many countries now have something similar. Governments should ensure these programs remain well-funded and perhaps expand them in biotech fields that require larger capital (for example, some advocate for SBIR Phase IIB matching grants up to $3-5M for biomedical companies to help push into clinical trials). Canada's NRC-IRAP is one such bridge program that could be scaled up. Europe's new European Innovation Council (EIC) fund is another model, it not only gives grants but can take equity in high-risk startups to support them through development. These kinds of public venture funds can be game-changers, as Germany saw when it co-invested with private VCs in the 90s. The private sector is more willing to invest when the government shares risk.
Prioritizing Key Stages like Clinical Trials: Especially in biotech/health, late-stage clinical trials are hugely expensive and often beyond the reach of startups or even mid-size firms. If governments want local innovations to reach patients, helping fund domestic trials is crucial. As one commentary noted, "without strong investment in [clinical trials], Canadian innovations stall before they can be commercialized". This doesn't mean governments should fund full Phase III trials for any drug candidate, but strategic coinvestment or infrastructure (like national clinical trial networks that startups can utilize at lower cost) can make a difference. During the COVID-19 pandemic, we saw accelerated models of this, with states funding vaccine trials or manufacturing capacity in advance. Those models can be applied in peacetime to other high-need areas (e.g., new antibiotics, where market incentives are weak but societal need is high). In Singapore, the state created Therapeutics Development Review committees to identify the most promising research and push it to clinic with targeted funds, ensuring promising science doesn't die on the vine due to lack of trial data.
Regional and International Collaboration: Often a single country, even the U.S., cannot single-handedly take every good idea to market. International funding collaborations (like EU's Horizon programs, or US-Canada joint initiatives) allow sharing the load and pooling expertise. Governments should encourage their researchers to engage in such collaborations which often come with commercialization support. For example, EU's Horizon 2020, with a €75.6B budget, explicitly tied research funding to innovation outcomes and is projected to deliver a 5x return by 2040 through spurring private investment and new products. The lesson: a well-funded, impact-oriented program can pay back multiples in economic and health benefits.
In summary, government funding bodies hold the keys to either leave innovations stranded at the patent stage or guide them forward. Singh and Chen's call to action, that funding agencies "really focus on the impact", resonates strongly here. By tweaking policies and launching translation-focused initiatives, governments can turn more biotech patents into actual cures, cleaner technologies, and biotech-based industries at home.
Frameworks and Models That Accelerate Commercialization
Beyond government grants and policies, there are structural frameworks and models that have proven effective in closing the commercialization gap. Understanding these can help stakeholders replicate success:
Bayh-Dole Act (United States): This legislation is often cited as the turning point that unlocked academic commercialization in the U.S. Before 1980, inventions from federally funded research were largely government-owned and seldom licensed (as noted earlier, only a few percent were commercialized). Bayh-Dole allowed universities and nonprofits to own the IP arising from research they conduct with federal funds, provided they file patents and seek to commercialize. The result was an explosion of university tech-transfer offices and licensing deals, by 2014, U.S. universities were securing over 6,300 licenses a year and had filed 23,000+ patents. Cancer drugs, vaccines, biotech tools like CRISPR, many have roots in academic labs that leveraged Bayh-Dole to spin out companies or license to existing firms. Framework impact: Other countries took note. The U.K., as mentioned, changed IP rules in 1985 to empower universities, and countries like Japan, China, and many in Europe adopted "Bayh-Dole-like" laws in the 2000s. However, legislation alone isn't enough, it must be coupled with capacity (tech transfer staff, funding, etc.). Bayh-Dole's success was amplified by the entrepreneurial culture and capital in places like Silicon Valley and Boston. Still, as a framework, it's a cornerstone: clear IP ownership + obligations to commercialize = more chances for impact.
Small Business Innovation Research (SBIR) Program: SBIR (and the related STTR for research institutions) provides a framework for funding high-risk, high-impact projects through small companies. Its multi-phase structure (feasibility, development, then commercialization with private funds) forces a focus on practical application from the get-go. A National Academy of Sciences review found SBIR "effective in stimulating technological innovation and increasing private sector commercialization of federally funded R&D". Importantly, SBIR set a template others emulate, the U.K. created SBRI, the EU has SME Instrument/EIC, etc., all aiming to engage small innovators in national R&D goals. An often overlooked feature is that SBIR topics are tied to agency needs (e.g., NIH might call for new cancer diagnostics), so from day one, a startup is working on a real-world problem with a potential built-in customer. Lesson: A structured program that marries funding with market need and mentorship can yield higher commercialization rates than untargeted research grants.
Public-Private Partnerships and Consortia: Biotech advancements often require collaboration (e.g., a new drug needs input from chemists, biologists, clinicians, manufacturers). Frameworks that bring together academia, industry, and government in consortia can accelerate progress. For example, the U.K.'s Catapult model provides a neutral innovation space with industry-scale equipment where academia and companies co-develop technology. Germany's BioRegions fostered networks of universities, startups, and larger companies in regions like Munich and Heidelberg, which created local ecosystems where ideas could more easily turn into companies with the right support. In the U.S., initiatives like the Manufacturing USA institutes or NIH's Accelerating Medicines Partnership (with pharma companies) create pre-competitive collaboration frameworks, sharing costs and expertise to de-risk new technologies. These models show that ecosystem-building, not just individual project funding, is vital. If you have a cluster with venture investors, mentors, lawyers, talent, and facilities, a startup's odds of success go up significantly. As Kevin Chen emphasized, "get to know your industry" and use available resources, clusters and partnerships make such resources accessible to newcomers.
Translational Accelerator Programs: In recent years, we've seen the rise of accelerators specifically for biotech (akin to Y Combinator in software). IndieBio, for instance, provides a framework of a fixed-term, cohort-based mentorship and seed funding for bio startups. These accelerators push academic founders to iterate on business models and prototypes quickly, often achieving in 4-6 months what might otherwise take years. Governments and universities are also launching their own: e.g., Stanford's SPARK program mentors researchers in drug development and has led to dozens of clinical trials; Canada's Lab2Market is a national program educating grad students and professors on commercialization. The structured approach and education-focused framework help change mindsets, scientists start to think like entrepreneur-innovators, which is exactly the cultural shift needed to bridge the gap.
Patent and Market Incentives: Frameworks like Patent Box (lower taxes on income from IP) in the U.K., Netherlands, Belgium, and being considered in Canada, aim to tilt the economics in favor of local commercialization. While not a silver bullet, early evidence from the U.K. Patent Box suggests an increase in patenting and perhaps retention of IP-intensive businesses. Another model is advanced market commitments, essentially guaranteeing a market if a product is developed (famously used for pneumococcal vaccines globally, and more recently for COVID vaccines by some governments). If, say, a government commits to buy a certain amount of a new antibiotic once approved, that promise itself is a framework that encourages companies to invest in development that otherwise might seem unprofitable. Prizes and challenges (like XPRIZE or government innovation prizes) also create a goal-oriented path to commercialization for specific needs (e.g., a prize for a rapid Ebola test spurred multiple viable products). These incentive frameworks ensure that if a researcher or startup delivers a solution, the reward (market or financial) is clearly in place, thus bridging the gap between solving a problem and finding a customer.
In essence, there is no one-size-fits-all model, but the combinations of policy (Bayh-Dole), funding (SBIR, grants), collaborative spaces (clusters, catapults), and incentives (tax, market promises) form a toolkit that, if well implemented, dramatically increases the conversion of biotech innovation into societal impact. Singh and Chen's insights align with this: they seek a holistic ecosystem where government, academia, and industry each play their part to ensure great science doesn't end at a patent but rather at a product on the shelf helping people.
Closing the Gap: Strategies for Stakeholders
Bridging the gap between biotech patents and commercialization is a multi-stakeholder effort. Governments, startups (and their academic founders), and investors each have a role in closing this divide. Drawing on the analysis above and the podcast insights, here are actionable strategies for each:
For Governments and Policymakers: Make commercialization a policy priority. This means funding translational research and early product development (not just basic research), measuring success by real-world impact (products launched, startups scaled, jobs created) in addition to publications. Expand or create SBIR-like programs and innovation funds to de-risk early stages. Implement incentives such as patent boxes or matching investments to keep IP-rich companies domestic. Streamline regulatory pathways for innovative products (for instance, fast-track review for breakthrough therapies) to shorten time-to-market. Finally, invest in infrastructure, from incubators and biomanufacturing facilities to talent development, that forms the backbone of a thriving biotech ecosystem.
For Biotech Startups and Academic Entrepreneurs: Embrace an entrepreneurial mindset early. As Kevin Chen advises, leverage available resources and "use what's out there" before leaping, for example, utilize university incubators, apply for grant programs, collaborate with industry mentors. Protect your IP strategically but remain flexible (sometimes a partnership or licensing deal can bring your innovation to market faster than going it alone). Build interdisciplinary teams, pair scientists with experienced business leads early, recognizing that biotech is a team sport requiring diverse skills. Plan for the long haul by plotting out the path from prototype to patient: identify technical milestones, regulatory requirements, and cost estimates. This will help in pitching for funding and also in de-risking step by step. Importantly, validate the market need for your innovation by engaging with customers (hospitals, pharma, end-users) early, a brilliant invention is only valuable if it solves a real problem that someone is willing to pay for.
For Investors (VCs, Angels, Corporate Venture): Develop a keen understanding of biotech timelines and value inflection points. Biotech isn't software; early due diligence should assess not just the science, but the plan for reaching key milestones (e.g., an IND filing or a prototype device clearance) that prove commercial viability. Be willing to provide patient capital or creative financing structures (like milestone-based investments) that align with these long development cycles. Partner with government programs when possible, e.g., co-invest alongside public funds that absorb first loss, to spread risk. Also, engage with academia proactively: programs like accelerators, mentoring networks or even adjunct roles can give investors a first look at promising technologies and a chance to guide them toward market needs. By being hands-on and supportive (rather than just chasing the next hype), investors can both find great opportunities and materially help startups bridge the gap. In regions with less developed ecosystems, investors could lobby for policy supports (many VCs, for instance, support the creation of patent boxes and funds as it ultimately increases deal flow and success rates).
For Research Institutions and Universities: Encourage and reward innovation translation. Universities should promote entrepreneurial leave schemes (like Singapore's A*STAR did) so faculty can attempt startups without derailing their academic career. Tech-transfer offices need adequate funding and talent, perhaps bringing in experienced industry people, to scout inventions and manage them professionally. Embed commercialization into the training of scientists (offer courses on biotech entrepreneurship, IP law, regulatory science). Create proof-of-concept grant programs on campus that give researchers small amounts of money to do experiments that validate market potential (many schools now have "gap funds" or innovation grants for this purpose). Celebrate the successes, when a faculty startup raises funding or a product from the university reaches market, make it visible. Culture matters: if young scientists see peers succeeding in startups, they will be inspired to try. Conversely, remove any stigma that might exist around leaving academia for industry, making it clear that such paths are valued outcomes of the university's mission to impact society.
For Industry and Corporate Partners: Large pharma and biotech companies can be pivotal in bridging the gap by adopting a open innovation mindset. This means actively licensing in early-stage technologies, collaborating with academia on research and early trials, and providing expertise or incubator space to startups. Corporate venture arms can invest in startups not only for financial return but to nurture strategic areas of interest, effectively mentoring them through development. Companies can also advocate for better funding of science translation (as stakeholders, they benefit from a stronger pipeline of innovations). Additionally, where feasible, being the first customer or beta-tester for a startup's product (for example, a big agri-tech company piloting a biotech crop technology from a startup) can validate the startup and help it attract further investment. By extending their R&D reach through startups, corporations both fill their innovation pipeline and help emergent companies survive the early years.
Key Takeaways and Action Points
Bridging the gap between biotech patents and commercialization is crucial for translating scientific brilliance into societal and economic value. The disconnect is real, but not insurmountable. Structurally, we need frameworks that align research with industry, and financially, we need smart capital at the right stages. Governments, entrepreneurs, and investors must collaborate to ensure that patents don't just add to CVs but lead to new medicines, sustainable materials, and biotech solutions that improve lives. As Guru Singh summed up the vision: it's about "turning groundbreaking science into tangible solutions". Below are actionable steps for each stakeholder group to help close this gap:
Policymakers: Shift research funding strategies to emphasize impact. For example, expand translational grant programs (similar to SBIR) and consider tax incentives like patent boxes to encourage domestic commercialization. Invest in shared infrastructure (incubators, trial networks) that lowers the barrier for startups to progress. Track success not by patents alone but by products launched and scale-ups grown.
Universities and Labs: Build a pro-commercialization culture. Establish easy IP policies and support systems for spin-off companies (seed funds, mentorship, sabbaticals for startup founders). Integrate entrepreneurship training for scientists. Reward faculty and students for patents that lead to licenses or startups, on par with publications, to signal that real-world impact is valued.
Biotech Entrepreneurs: Start with the end in mind. Engage early with end-users and regulators to shape your invention into a viable product. Leverage non-dilutive funding and incubators to de-risk your startup before seeking large investments. Protect your IP smartly, but be open to partnerships that can propel development. Assemble a diverse team, scientific talent plus experienced business and regulatory advisors, to navigate the complex journey to market.
Investors: Embrace a long-term outlook and domain expertise in biotech. Use creative financing (tranche investments, alliance with public grants) to support companies through lengthy R&D phases. Mentor and coach scientific founders, often your guidance can be as valuable as your capital in shaping a fundable business. Advocate for and utilize government co-funding programs to amplify the impact of your investments.
Global Collaboration: All stakeholders should look beyond silos, collaborate across borders and sectors. Participate in international initiatives or cross-industry consortia tackling big challenges (cancer, climate biotech, pandemics). Such collaboration can provide resources and knowledge that single entities lack, accelerating commercialization. Remember that biotech innovation is an ecosystem play: a discovery in a university, backed by a government grant, nurtured by an accelerator, financed by a VC, and partnered with an established company, can together achieve what none could alone.
By executing on these strategies, the biotech sector can significantly narrow the gap between invention and impact. The goal is that a brilliant idea in the lab doesn't remain an idea or a patent on a shelf, instead, it becomes a product that saves lives or improves our world, fulfilling the true promise of biotechnology. Bridging this gap is challenging, but as the leaders on talk is biotech! remind us, it is both necessary and achievable with concerted effort and the right support structures. The next insulin, the next mRNA vaccine, or the next sustainable bioplastic shouldn't have to leave its home country to succeed, with the gap bridged, it can be developed and delivered right where it was discovered, to the benefit of all.
Comentarios